Single Entity Electrochemistry
The stochastic collisions of individual suspended nanoparticles (NPs) with a potentiostated, inert working electrode provide a simple, ultrasensitive and high throughput tool to analyze and characterize single NPs and/or ongoing reactions on them. We develop and apply these so-called “nano impact” studies in a variety of contexts.
Electrocatalysis studies
During its contact at the electrode, the “wired” nanoparticle can act as a nanoelectrode and catalyze a redox reaction. Due to the highly efficient mass transport at the nanoscale, extremely high current densities can be obtained. This provides an efficient route to gain insights into the catalytic properties of a (new) nanomaterial, including both intrinsic reaction mechanism and kinetics. For example, nano impact studies on the oxygen evolution reaction (OER) at ca. 4 nm sized CoFe2O4 spinel nanoparticles result characteristic, potential-dependent current steps.[1] At high overpotentials, the step height is directly proportional to the distribution of the particle size and O2 (product) concentration in the solution, revealing that diffusion of produced O2 away from the catalyst surface is the limiting factor. Accordingly, nano impact studies in Ar saturated electrolyte show distinct signals in the presence of the catalyst, while current steps recorded in air are smaller than those recorded in the absence of O2 in the electrolyte and no current step can be observed in O2 saturated electrolyte.
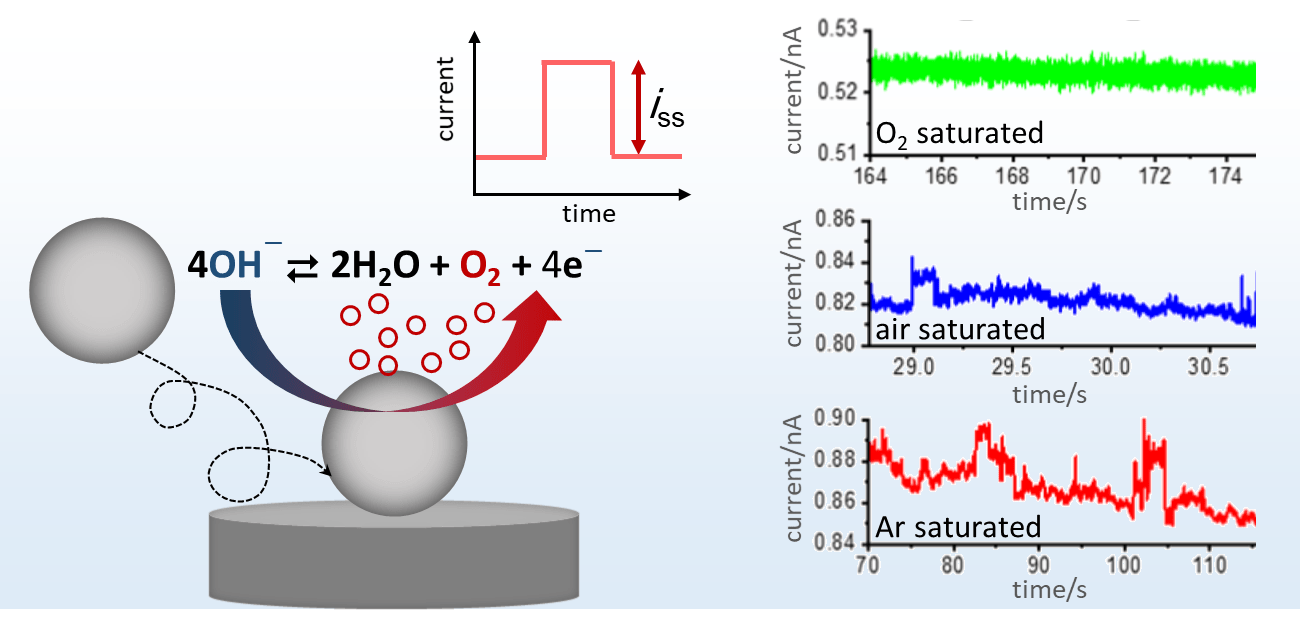
Investigation of electrical double-layer (EDL)
During nano impact events, the EDL of the colliding nanoparticle is perturbed. The resulting (dis)charging process gives rise to a transient current-time event (called blip or spike) that can be analyzed to gain direct insights into the electronic properties of the impacting particle. As the EDL rearrangement is measured while the collision occurs, information on colloidal nanoparticles can be obtained (e. g. their potential in solution), instead of being limited to usual surface-immobilized nanoparticles. Capacitive impacts of platinum NPs reveals an EDL capacitance much higher than that predicted by classical EDL models, which omit the influence of metal-water interactions.[2] Such interactions can lead to ion accumulation and water chemisorption at the metal surface providing a much higher charge storage ability at the interface.
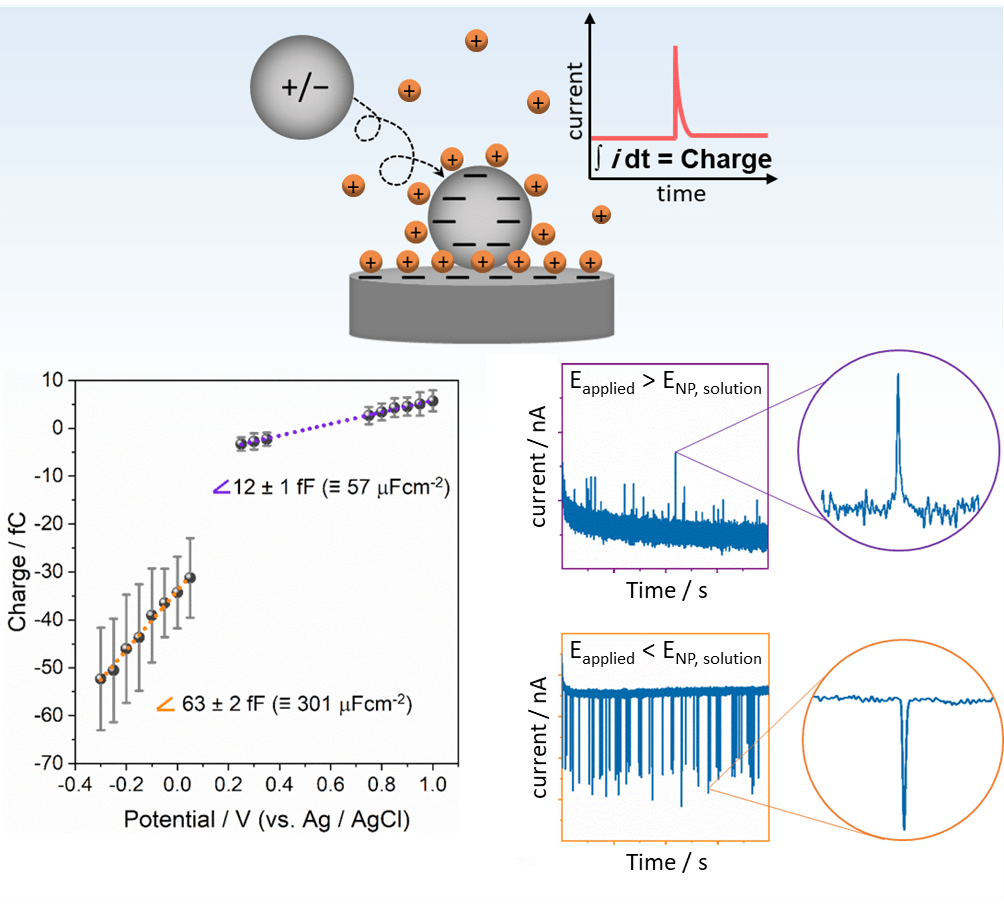
Studying battery materials
Nano impact electrochemistry also enables us to study active alkali-ion insertion materials for battery applications at the single-particle level. The current signal associated with each nanoparticle collision provides mechanistic information, e. g. about the rate-limiting step of the charging/discharging process in a battery. For instance, nano impact experiments of individual TiO2 particles in non-aqueous media reveal that their intrinsic lithiation rate is not limited by interfacial ion transfer kinetics, mobility of ion and/or electrons in the bulk of the particle, but by the solid-solid electron transfer.[3]
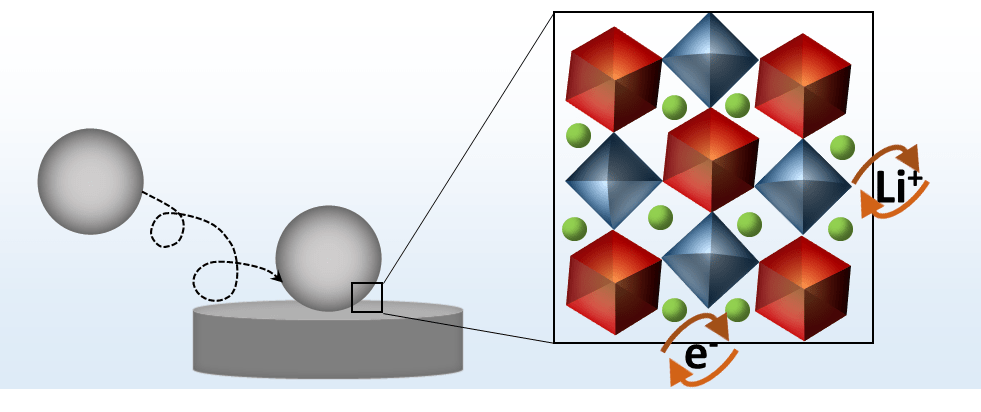
Electrochemical Characterization of Nanoparticles
By electron transfer between the colliding NP and a suitably polarized electrode, oxidation or reduction of the colliding particle can occur. The electrochemical conversion of individual nanoparticles results in transient current responses revealing their physical properties, e.g., size, composition, structural arrangement (core-shell vs. alloy), agglomeration state, and/or their concentration and the associated electrode kinetics. For instance, bimetallic NPs can be characterized to extract information about particle size and the ratio of the two components. By comparing the electrical charge of current peaks at different applied potentials, we are able to derive the size and the component ratio of the bimetallic NP. At a moderate potential, only the less-noble component of the NP is oxidized, while, both components of the NP react at high potentials. The ratio of both peak charges reveals the content of the less noble component. For instance, nano impact analysis on 14 nm sized Ag0.73Au0.27 alloy nanoparticles at different potentials were shown to yield the size and composition of individual NPs.[4] This approach is expected to give valuable insights into particle-to-particle differences in electrochemical dealloying, which is a great tool to improve the catalytic activity of NPs by providing the desired porosity and composition.
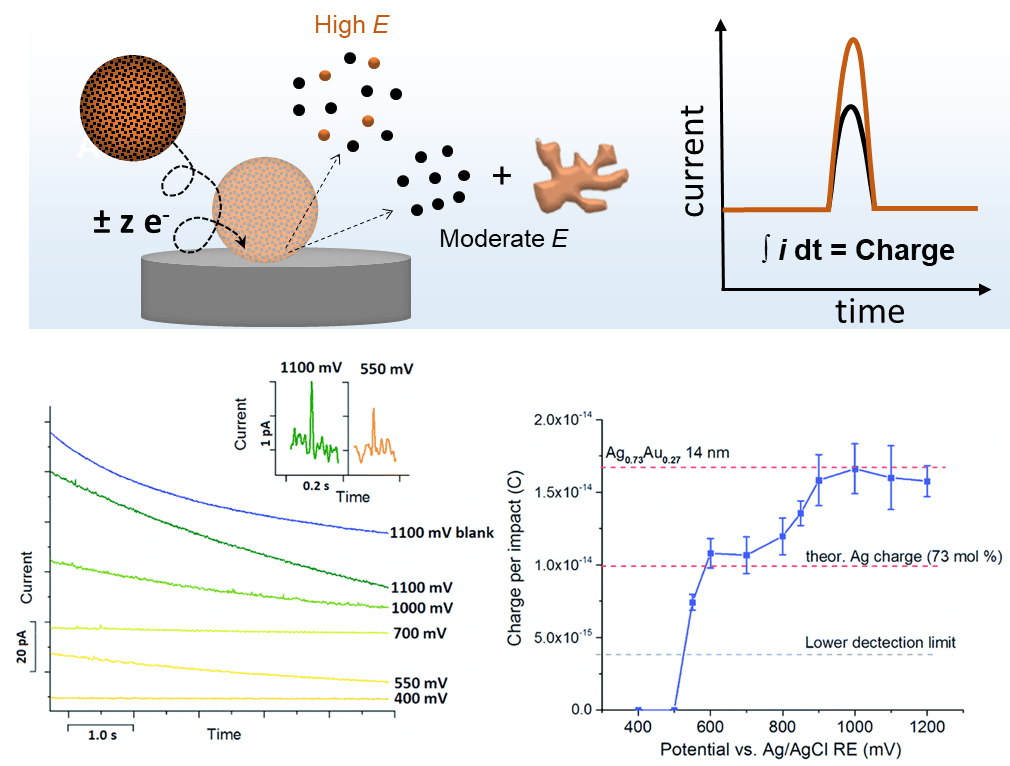
As we elucidated in our group by combining electrochemistry with identical location electron microscopy for AgAu alloy NP ensembles and the hydrogen evolution reaction as a model system, morphological and compositional changes of the NPs induced by dealloying, can considerably improve the electrocatalytic properties of nanocatalysts.[5]
[1] A. El Arrassi, Z. Liu, M. V. Evers, N. Blanc, G. Bendt, S. Saddeler, D. Tetzlaff, D. Pohl, C. Damm, S. Schulz, K. Tschulik, J. Am. Chem. Soc. 2019, 141, 23, 9197.
[2] M. A. Sani, N. G. Pavlopoulos, S. Pezzotti, A. Serva, P. Cignoni, J. Linnemann, M. Salanne, M. P. Gaigeot, Kristina Tschulik, Angew. Chem. Int. Ed. 2022, 61, e202112679.
[3] T. Löffler, J. Clausmeyer, P. Wilde, K. Tschulik, W. Schuhmann, E. Ventosa, Nano Energy 2019, 57, 827.
[4] E. N. Saw, V. Grasmik, C. Rurainsky, M. Epple, K. Tschulik, Faraday discuss. 2016, 193, 327.
[5] C. Rurainsky, A. G. Manjón, F. Hiege, Y. T. Chen, C. Scheu, K. Tschulik, J. Mater. Chem. A 2020, 8, 37, 19405.